Full episode transcript below. Beware of typos!
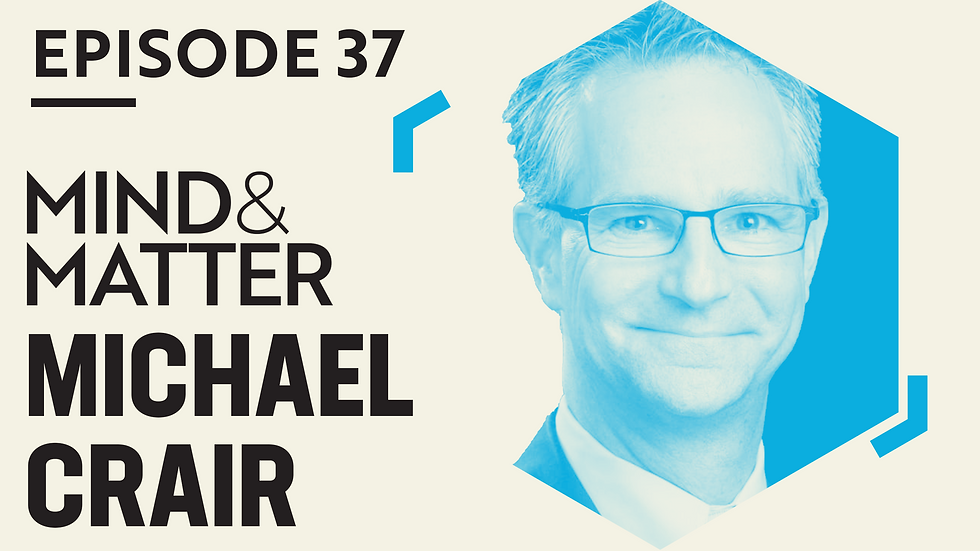
Nick Jikomes
Professor Michael Crair, thank you for joining me.
Michael Crair 4:51
Cheers. It's nice to be with you, Nick.
Nick Jikomes 4:55
Can you describe for everyone who you are and where you're calling in from today?
Michael Crair 4:59
Yeah, you So my name is Mike Mike Crair. I'm a professor in the Department of Neuroscience at Yale University. I'm also the Vice Provost for Research at Yale, and calling from Guilford, Connecticut, which is a little suburb of New Haven, Connecticut, where Yale is located.
Nick Jikomes 5:15
So what do you study as a neuroscientist?
Michael Crair 5:18
I'm interested in brain development, and sort of fundamentally curious in trying to understand how the brain wires itself up during development. And that's a pretty big topic. It's it's a diverse topic. But it's, I think it's a fundamental fundamental interest to just understanding brain function is to try to understand how we all get wired up over the course of development.
Nick Jikomes 5:42
What's What's your sort of ultimate aspiration? Is a question, why are you trying to answer the kinds of questions that you are?
Michael Crair 5:50
Yeah, so of course, it's a good question. I'm interested in how we think. Right, I want to understand how we think and that's a $6 million question to use an old phrase, that's a hard one. And I think, fundamentally, what I decided was, at least one reasonable way to try to understand how we think is to figure out how the brain gets put together in the first place, if we can understand sort of the mechanisms for wiring up the brain that will give us fundamental insight into how it is that the brain works, how we think. And, you know, I do that do those studies by focusing on sensory systems, the visual system, in particular, because it's a relatively simple system to understand how the brain wiring occurs over the course of development. So I understand how the brain works very deep, fundamental question. And I choose to study trying to understand how it gets wired up as a as a as the best entree into that fundamental question, how do we think?
Nick Jikomes 6:52
Yeah, I want to talk a little bit about some of the structures in the parts of the brain that your lab studies on your lab website, you've got the research overview page, and it says something like, the brains of animals in the brains of animals, the sensory world is organized in regular neuronal arrays or maps. So what exactly are these sensory maps in the brain?
Michael Crair 7:18
Yeah, so the easiest way to think about it is, at least one simple thing, a simple way that many people can grasp easily is the map of the body surface. So in the brain, you have areas that respond to stimulation of the body surface, the sensory periphery, and neighboring neurons in the brain will respond to the stimulation of neighboring sensory receptors on the body surface. And so this is a basically a map of the body in the brain. So it's in the fundamental feature of those maps is that there is a preservation of neighborhood relationships. That is, neighboring sensory receptors are mapped to neighboring locations in the brain. That's a somatosensory system, analog of a map, a sensory map, the same sort of map occurs in the visual system, where neighboring positions in the visual world are mapped to neighboring locations in the brain. So it's, it's a sort of morphed map of the visual world, in the brain, where neighborhood relationships are preserved. That's what we mean by maps.
Nick Jikomes 8:24
I see. So So morphed map is that why when people see that image of the so called somatosensory homunculus, yeah, looks like a very warped human being with exaggerated features for some places.
Michael Crair 8:36
Yeah, exactly. That's exactly right. So and the the morphed features represent basically the density of receptors on the sensory periphery. So those parts of the sensory periphery, like the, like, your fingertips have lots and lots of receptors. So there's a dramatically enlarged representation of your fingertips in the brain. And in the visual system, there's an analogous morphing, where the fovea, the center of your vision has a very, very high density of photoreceptors. And so there's a dramatically enlarged representation or, or piece of the visual cortex, which represents or responds to stimulation of just the sense the center of your vision, the fovea. So it's a morphed map, so that the distances are not preserved, but neighborhood relationships are preserved.
Nick Jikomes 9:23
So I'm going to ask a question that's, that's maybe vague, but I do think it's interesting and important. Why would animal brains be organized in this kind of way? Are there other? Are there other like bio physically plausible ways you could imagine the brain being organized and why did evolution create this kind of structure with these kinds of maps?
Michael Crair 9:45
So I think these maps are really just the starting point of neural computations that lead to say, cognition, higher order cognition. It's a It's sort of a basic building block or representation of the sensory periphery, that, you know, a fundamental feature of the world are these neighborhood relationships, like neighboring locations on your fingertip or neighboring locations in the visual world. And so one of the initial roles of the sensory system in the brain is just to take the input from the sensory system and code it in a way, which is then easily digestible for higher order processing. So these these maps are robust in the initial stages of neural processing, and then they become quite complex and not obvious. And the the mapping relations break down. So I think it's an efficient way of coding information about the sensory periphery. That's why they're common.
Nick Jikomes 10:50
A lot of the questions that I want to get at next will have to do with random, and, you know, the extent to which structures in the brain such as these maps are self organized, and, and just sort of an unfolding of things intrinsically specified by the genome, versus things that are shaped by experience, and that are very malleable. So before we get there, let's start off by talking plasticity, generally speaking, so what is plasticity? And what are some good examples? In the mammalian brain of plasticity?
Michael Crair 11:23
Yeah, okay. So the plasticity is the malleability, or the, or the ability of the brain to change its response, or, in this case, in the case of maps, the representation of the sensory world based upon the pattern of sensory input. So we're talking about, you know, maps of representation of, say, the body surface into the brain, that map is not fixed, that map can change over time, especially it can change dramatically during development. And so plasticity is that would be the changing of the wiring, that would lead to a difference in the representation of the sensory world. So for instance, if you, for some reason are, lose a finger during early development, so that you know your middle finger, let's say you're in an accident, you lose the middle finger, you would no longer have input from that finger into the brain. And the map representing your head would change over time, it's plastic, such that the two remaining neighboring fingers would come to be represented in neighboring locations in the brain, and the middle finger would no longer be represented, it would disappear. That sort of plasticity occurs in spades. early in development, it's a remapping or rewiring of the brain to accurately represent the condition of the sensory periphery. It that sort of plasticity is, as I said, very, very robust, early in development, and much more subdued, much more modest in scale, as you get older and older, such that the maps become relatively fixed as an adult.
Nick Jikomes 13:02
And what's the purpose of plasticity being at high levels basically, earlier on in development, and then going away, you often hear especially in the news, plasticity used as a very positive, it's always good. Is that is that the case? And does that have anything to do with why plasticity sort of wings over time? So, you know,
Michael Crair 13:23
the honest answer is, we don't know. I can give you my hypothesis or my understanding of why why the plasticity wanes over time. So what what appears to be the case is that the plasticity wanes. So the plasticity remains robust in the adult in, in higher order cognitive areas, like higher order, cognition is a very plot, the brain circuits responsible for cognition are very plastic in the in the adult. And that's what allows us to think and learn as adults. The the plasticity is wanes in representations of the sensory periphery, the sort of input circuits, and then as we get higher up in the sensory and computational hierarchy in the brain, the plasticity is preserved in the adult, so the further you are in the sensory periphery, the earlier in development, that plasticity wanes, and the circuits become fixed. So it seems to cascade from the outside in. So the plasticity remains robust in the adult for cognitive circuits. But in the butt as you work your way out to the sensory periphery, the plasticity gets less and less, and the surface become more and more crystallized earlier in earlier development. So that's, it seems to be a common rule. The question is, why would that why would that be I surmise that you're you're like you're the neural circuit. You're like building a cognitive circuit, a cognitive house, and the representation of the sensory world these maps are The foundation, you need to, you need to have a representation of the world. And that provides a foundation for higher order cognitive, you know, thinking extraction of relationships and features of the world. And so you initially build the foundation, it's a little plastic as the foundation is developing and, and being born and then it becomes fixed. So that the higher order circuits can faithfully rely on the representation, which was built early in development of this external world. So I view it as a as the those those early plasticity is to build the right foundation, that foundation becomes fixed. And then the higher order circuits, which were are very plastic, remain very plastic, rely on a fixed foundation. So it knows what the century world representation is, it needs some sort of scaffold some structure to trust that is that is that it, you know, that is fixed for the higher order representations to, to then act on?
Nick Jikomes 16:06
Interesting, yeah, that makes us? Yeah, that makes a lot of sense.
Michael Crair 16:10
Yeah. And that's, you know, that's just a hypothesis, the way I, I conceptualize why there's plasticity, why that plasticity wanes over time, and it wanes more in some circuits than others, it clearly, the plasticity wanes earlier and more dramatically in the more peripheral circuits. And then as you work your way up in the brain, in this sort of cognitive architecture, the plasticity sustained higher and longer in the adult,
Nick Jikomes 16:38
I see. So if you're looking at a pair of neurons in the brain, the level of plasticity will tend to depend on a, how far into development you are, how old the animal is, and be how close to your eyes and your ears and the external world that circuit is, the further in the frustrating in this cognitive hierarchy you are, the more plastic the neurons will tend to be later.
Michael Crair 17:06
That's correct. That's right. And it's actually true in reverse to as you're working your way out to the motor system, that the, you know, the neural control of muscles tends to be more fixed. But the motor, the motor circuits in the higher order brain are more plastic in their representations and their abilities to adjust their control of the of the motor neurons. So it's sort of on the way in and also on the way out.
Nick Jikomes 17:32
So even though there's quite a bit of plasticity, even the sensory maps earlier, earlier on development, there is my understanding is that there's a lot of self organization that happens basically a lot that's pre specified in a coded in the genome, to what extent is that true in the sensor systems that you work on? And why would you want to have a fair amount of a sensory map or a structure in the brain, sort of pre pre coded and embedded in the genome? Ready to go?
Michael Crair 18:01
Yeah, so this is the kind of, you know, one of those major fundamental questions is, to what extent do you pre code the circuit in the genome? And to what extent do you allow activity plasticity to self organize the circuit, and this is phrased in various ways, and, you know, in different contexts, it's a little bit like the nature versus nurture debate, where nature would be the genome coding the circuit structure, and nurture being activity, shaping what the what the, what circuit forms based upon experience or the environment. And, you know, as is always the case, it's neither entirely one nor entirely, whether it's both nature or nurture, that shapes the development of the circuits. And I'm, my background is a physicist, I'm trained as a physicist, I got my bachelor's and PhD in physics from UC Berkeley and my PhD was studying dynamics, nonlinear dynamics. And there is a kind of intrinsic structure of dynamical systems have these intrinsic structure, which is self organizing. And, you know, in chaos theory, you talk about attractor networks and attractor states, and there's sort of the, the, the, the forces or nature of those systems lead to order in an internal self organizing way, a self organizing system. And so I was attracted to try to understand how the brain works. Insofar as it develops as a self organizing system. It has analogies to self organizing systems that have been studied in in physics. And so when I learned about plasticity in the developing brain as a graduate student, I realized there was a certain parallel to these self organizing systems in physics, where the plasticity and the role of sensory experience in shaping the connections would lead to accurate and efficient representations could be accurate and efficient representations of the sensory world as a as a computational convenience. And so it is absolutely the case is a long winded answer. But it's absolutely the case that both genes and the environment are important in the development of the nervous system. I'm less interested in the role that genes play. Because I don't think they really tell us about how the brain functions. I'm much more interested in how activity sensory experience shapes the development of the circuits, because that's self organization, I think gives you fundamental clues about brain function, how is it the brain organizes itself, it's a self organizing system really teaches you about how the brain works. Whereas the genes, the genes or the genes, they doesn't really tell you about function.
Nick Jikomes 21:10
Interesting. One of the interesting phenomena in the brain, that a lot of people may not be aware of, is the idea that neurons are spontaneously active, everyone, I think, has an intuitive understanding that, you know, when something happened, eyeballs see something, neurons in the in the visual system laid out with some kind of pattern. But even in the complete absence of sensory information, neurons are active, can you describe what spontaneous activity is and why it's maybe interesting to think about in terms of self organization?
Michael Crair 21:45
Sure. So one of the one of my earliest discoveries and this discovery is paid by colleagues in the field was that much of the brain appears to self brain circuits in the visual system, again, is where we've studied this in a greatest detail, appears to self organize, before vision, that is many of the fundamental circuits that are responsible for representing the visual world like location of stimuli and space, or orientation of edges or direction of movement of objects in space, the circuits that are responsible for that specific those specific response properties to location and direction and orientation, form, before vision before the animal has seen. And so that, that suggested that there must be genetic mechanisms that are responsible for the formation of the circuits, or that there was activity in the system that was present prior division that helped the circuit reorganized, and again, me and colleagues demonstrated the presence of this early spontaneous activity activity, which is actually generated in the nervous system, prior to vision that helps organize or train the circuit to form these sophisticated circuits for locating the location of objects or the direction of motion of objects in visual space. So it's an activity that's intrinsic to the nervous system that's not stimulated by outside light. And that early activity, this spontaneous activity is important for developing particular circuits in the brain. And I an analogy, which I think is apt and appropriate is the kind of activity dreaming activity what I what I refer is to dreaming, nobody is surprised or shocked that everybody dreams essentially, that dreaming activities, spontaneous activity in your dream state, you go to sleep, it's not. It's not dictated by sensory stimulus, it's activity which is generated in the brain, which resembles some you know, some activity you had in the during the day, it might reflect some experience you had in the past, or it might not it might be completely imaginary and fictitious, but it's a pattern activity in the brain which gives the some sense, you know, some sensation some has typically visual properties or might have olfactory or somatosensory properties. This is spontaneous activity in the brain in the adult brain. The developing brain has spontaneous activity to it has its own intrinsic pattern of activity, which we and others have shown is important in the wiring of the brain itself. The self organization of the brain during development is actually dependent upon this early spontaneous activity. That's completely separate from Senator experience.
Nick Jikomes 24:52
Interesting. Michael, I'm gonna pause real quick So, so that's interesting, what you're saying is, you know, we all dream every night. So we actually have direct experience of this kind of thing, the brain can simulate the world, even when it's not receiving inputs from the world. On the other hand, you know, I can imagine, like, you know, when I go to sleep tonight, I'm going to dream, these kinds of simulations will be, you know, playing some story in my in my mind's eye. But I could also imagine that, you know, even though the sensory input has been turned down, while I'm sleeping, all of it's been informed by previous experiences I had while waking. And so in some sense, maybe the information got in there from the outside world, and I retained it. But you've also done research showing that this type of thing happens, even in the developing system of a mouse before the mouse is ever even awake and opening its eyes in the world. So Right? Tell me if this is accurate, what you're saying basically, is, in mice, in the case of your lab, you can record from neurons in the visual system, or look at them using a microscope, record them using electrodes. And the patterns of activity that you see in the developing nervous system, before the mouse ever opens his eyes look, like they do with the mouse will later be opening its eyes and experiencing the world visually.
Michael Crair 26:20
So that that's not quite right, but it's close. So the difference is that, you know, it's hard to say, first of all, I, so let me back up a second, we definitely see activity in the young mouse before it opens his eyes, actually, before the retina is functional. So there's a robust, spontaneous activity in the developing eye and brain before vision is possible, the retina doesn't work. And what we another showed was that this early, what we refer to as spontaneous activity, that that's generated in the retina and propagates up to the brain is essential for the normal development of the visual circuits. So in the absence of this spontaneously generated, building, the inner neural circuits don't form correctly in the visual system. What we further showed, which is, I think, most remarkable is that this early spontaneous activity isn't random. It's not random firing of neurons in some kind of sort of salt and pepper fashion, or, you know, like fireworks or something like that it doesn't have a random, it's not random in its feature, but it's actually highly patterned. And the remarkable thing is that this early activity in the retina resembles the activity that would be present if the animal was running forward through space. So it's a pattern activity, which resembles the sensory experience, the animal would see if it was running through the forest forward in space, not turning around in a circle, not moving backwards, not stationary, but actually resembles the pattern of activity in the retina that would be generated in the retina, if the animal is running forward through space, and this pattern of activity propagates from the retina up into the brain circuits, including the lateral geniculate nucleus disappear clickless in the visual cortex, so all the way up the visual hierarchy in in mice. So I can't say whether the animal is sees something in a dreamlike state that resembles as what, you know, I can't interpret what the animal sees that it's, it looks like, if you it seems like to the animal like it's running forward through space, but I can't say is the stimulus, the activity that's generated in the retina, and that propagates up into the brain resembles the activity that would be present if the animal was running forward through space. So it's just it's an important distinction. It's a it's a little bit of a fine point. But I think it's an important distinction distinction, like I can't get in the brain of a mouse and know what it sees. But I can see activity patterns that are present, or similar to the activity patterns, it would see if it was running forward through space.
Nick Jikomes 29:31
So it's as if the brain is more or less simulating, the type of activity that's going to commonly come from visual experience in the world, which will often be is basically the idea that a common of moving through the world for the mouse is moving forward. And so that direction of motion is maybe the one that the brain is working on pre pre pre coding or pre working on before the animal actually ever sees anything.
Michael Crair 29:58
That's exactly right. And And I think it's, I think it's an evolutionary phenomenon. In other words, if you, you know, go back and evolution, animals like, you know, some primordial fish or amphibian, or whatever the primordial beast that the mammals came from, were, you know, swimming for through space are moving forward through space. And mammals have had a evolutionary adaptation that leads to very extended gestation. So they've, their, the time in the womb, is very long in comparison to say, and fish and frogs. And so, during this efficient frog, you know, hatches is a tadpole has a very primitive nervous system. And it continues to develop over many years, I mean, and many months. And the, that primitive nervous system has continuous wiring continuous formation of circuits from the eye to the brain, in the presence of visual stimulus. And for fish, or a tadpole, it's swimming forward through space. And so it's seeing stimulus come by it. And under normal circumstances, or in their primordial circumstances, the animal as its visual system was getting wired up, would have present this continuous or a relatively continuous stimulus moving forward through space, so the world coming past it. Now, since mammals have an extended gestation, they're obviously not seeing the world. That way, they actually the circuits are forming prior to the animal can see. And it's my hypothesis, that it's substituted the, the device, it's substituted a generation of spontaneous activity in the retina, to mimic the kind of activity it would have seen in the sort of primordial soup, as it was swimming forward through space. So it's, it's mimicking the stimulus, which was typical, of, of what an animal would see with a shorter gestation, earlier in evolution by this generation of these spontaneous patterns of activity. And that leads to the formation of circuits, which are appropriate for things like the detection of motion through space or neighborhood relationships, location of objects in the visual world. I hope that makes a little bit of sense, Nick.
Nick Jikomes 32:27
Yeah. Why would? Why would spontaneous activity be observed in neurons in the first place? Is this something that is baked in on purpose, quote, unquote, by evolution? Or is it and is it just sort of like, an animal inevitable, inevitable noise artifact that comes from being cells?
Michael Crair 32:49
Yeah, so um, I think cells neurons have this feature that they, they don't want to be silent. They, they have these what's referred to sort of homeostasis, they don't want to be too active, like firing all the time, and going crazy, but they don't want to be silent either. If they're firing at high rates all the time, then they're burning out. If they're completely silent, then they're not doing anything, they're not being useful. So they have a what appears to be a way to self regulate their activity. So to regulate, for instance, the ion channels in the membrane to maintain some basal level of activity. And so they're sort of spontaneously active naturally. And if you take away for instance, their input, they'll ramp up their intrinsic membrane properties, or the strength of their synapses to restore some level of activity. So there's a kind of homeostatic mechanism to maintain a happy level of activity. But what we're talking about here is much more than that, we're not talking about individual neuron neurons having a happy level of spontaneous activity. But actually, patterns of activity between large cohorts of neurons that are pattern, right, it's activity amongst large cohorts of neurons, which have specific spatial temporal patterns, not individual neurons, firing randomly with respect to each other. So the neurons themselves have this homeostatic tendency to be active. And then, in addition to that, in during development, what we showed is that there are communication mechanisms between the neurons that lead to coordinated patterns of activity, not just random firings of individual neurons.
Nick Jikomes 34:42
And so when you're doing these experiments in the lab, can you try and paint a visual picture for people of how you actually observed these patterns? What do the experiments actually look like?
Michael Crair 34:51
Yeah, so you know, we make use of modern optical imaging techniques and genetic expression techniques that allow us to visualize with light the activity of neurons. And, you know, the way that's done now commonly is you use gene expression techniques to make neurons express a green fluorescent protein, this protein that's derived from jellyfish originally, that actually is been modified to act as a activity sensor. It's called a G camp. Okay? It's a green fluorescent protein, which is linked to a calcium sensor, which is a measure of neural activity. So when the neurons are active, when they're firing action potentials, they glow brightly. And when they're inactive, when they're not firing action potentials, they do not glow brightly. So what we do is make the neurons of our interest in the visual system express this, this glowing protein GFP linked to the activity indicator G camp, so that when they're active, they fluoresce blight brightly. And with a microscope, by just actually, all we need to do simply is peel back the skin above the skull, because we can't see through the skin. But you can see through the skull to the brain, the fluorescence is so bright, that especially in young animals, you can see right through the skull to the brain. And when the neurons are active, they light up like a Christmas tree. And you can see the activity in with a microscope with a pretty standard simple microscope. So we're visualizing the spontaneous activity in the brain, through this optical technique, using your microscopes, and these genetically encoded activity indicators.
Nick Jikomes 36:59
So so when you say that you see these patterns of activity, that's a very literal statement, you literally have tricks that allow you to watch the neurons actually light up.
Michael Crair 37:07
That's right, that's exactly right. It's not just, you know, it's not a figurative see, it's a literal, see, they are fluorescing, They're glowing, when they're active. And we can see that glowing activity.
Nick Jikomes 37:23
So how do you think these paths also were sort of in the brain? are you observing this? Is it relatively close to the retina? Is it higher up? Is it? Is it on the sensory maps? Or where is it in the
Michael Crair 37:34
higher? Yes, right? It's one of the century mats actually, we can do these experiments anywhere that's accessible by light. Okay, so one of the challenges if you're, you know, deep in the brain, the light doesn't penetrate. And so you can't see the neurons or the, the activity. So anywhere that's accessible, by light, you can see and in, in our experiments, the typical structures, which we image is a structure called the visual cortex, the primary visual cortex, which is the entry point for a visual information into the cortex, the cognitive part of the mammalian brain. And another typical visual structure, which we will image This activity is called the superior colliculus, which sits at the roof of the midbrain, which is another important visual nucleus, in in mammals and vertebrates. So those are the two typical areas of the brain that we image, this activity, the superior colliculus, and in the visual cortex, and in both of these structures, there are maps of the visual world, so that for instance, neighboring neurons in the superior colliculus will respond to stimuli in neighboring locations in the visual world. So there's one of these morphed maps of the visual world in the superior colliculus, and in the visual cortex. So when we see activity in one location, the spirit clickers, we know that corresponds to what would happen if you were stimulating one location in the individual world, because of this map.
Nick Jikomes 39:05
So in some sense, you could watch the neurons light up in such an area and reconstruct what the animal might be seeing
Michael Crair 39:13
eye in some sense, but I hesitate to draw that I don't want to claim I can step into the mind of a mouse and see what it sees. What I can tell you the pattern of activity, it resembles the activity that would be present when you present this particular visual stimulus. So in some sense, you're right. But I hesitate to anthropomorphize too much.
Nick Jikomes 39:38
So to what extent do you think this type of phenomenon is happening in the human brain? Is it maybe happening less because we have a more extended childhood and were born in more of a state of helplessness compared to other animals, or is this likely happening to some extent in the human brain as well?
Michael Crair 39:55
I think it's happening in the human brain. So courts The kinds of experiments we're doing are virtually impossible to do in humans. Some neural activity recordings can be done in infants using techniques such as functional magnetic resonance imaging. But it doesn't have the resolution to be able to observe these spontaneous activity patterns that we can observe, observe in other mammals. So I actually don't know, for with any certainty whether it's happening in humans, what I can say is, spontaneous activity in the retina, happens in all other mammals for which it's been investigated. So non other non human primates, carnivores, and rodents, they all have this spontaneous activity early in development prior to vision. So I presume it's also happening in humans, but I can't say with certainty. So. So we know this early spontaneous activity exists. And the degree to which it's patterned in variety of mammalian species is a little bit uncertain. So the specific patterns that we observe we've observed in mice, we don't know whether this earliest activity that resembles moving forward through space is similar will be similar in other mammals. And that's something we need to find out. But we just we know for sure that there is early spontaneous activity, we just don't know what the details about the pattern and other mammals, and we actually know nothing about humans, specifically.
Nick Jikomes 41:38
So so far, we've established that all neurons are spontaneously active, in some sense. They're, they're intrinsically built, so that they're going to want to be spontaneously active at some rate, but not only are they spontaneously active, when you guys actually look at what they're doing. In the developing mouse brain, you see that the activity is patterned has some kind of pattern. And remarkably, the pattern looks, it looks very much like the patterns that you might see when the animals actually looking at something. How do you How does, how does the brain actually give it this pattern? What's what's physically happening, so that it knows how to create these specific patterns, and you don't just see like a random array of activity?
Michael Crair 42:20
Yeah, so that's a certainly one of the most fascinating things about this is it in order to understand these activity patterns and manipulate them change them, to be able to determine what their function is, what their role is brain circuit development, we had to, we had to understand the source and the risk. And you know what the system was responsible for generating the specific pattern that we see. So a lot of our work has been what I would call mechanistic work in dissecting the source and a method by which the activity has this pattern nature. And so we know a lot about it, I don't think we understand it completely, but we know a lot about it. So one of the most interesting things is that not only are neurons intrinsic, and do they like to be active, but there are specific neural connections in the developing retina, that form for the express purpose of generating these wave like activity patterns that resemble the animal moving forward through space. What I mean is that they're not random connections that formed during development. They're specific neural synapses connections in the developing retina, which form in a pattern to generate these waves that resemble forward motion through space, they're there, and they're developmentally controlled. So the circuits form for a specific epic during development for in mice, it's about a week, and then those circuits decay away, and the activity patterns change and the animal becomes has a visual function, so it responds to light instead of generating these pattern waves of activity. So there is a transient mechanism and changing circuit that's formed during development. We think specifically to generate these waves of activity that mimic this forward motion to space for the purpose of changing the visual system, so that it can see once the animal eventually does open its eyes. So it's a it's a developmental adaptation. With a specific time point during development, when the circuit forms and then dissipates and becomes something completely different.
Nick Jikomes 44:45
Is it possible to actually test an idea like that, where somehow you actually disrupt these patterns of activity before eye opening and then show that the animals will less and less able to perceive things like optic flow?
Michael Crair 45:00
Right. Yes, it is. And that's, you know, that's one of sort of an important technique is to test the, the role of these early spontaneous activity patterns, they're sort of what I would refer to as their causative role. If you disrupt these activity patterns, what happens to the development of the neural circuit, so there's a very coarse way a sort of Sledgehammer way, which is to eliminate the activity completely, just basically completely block it. And if you completely block this early spontaneous activity, then the neural circuits that form are very perturbed. The animals vision is very perturbed. It's, it's a sort of, we'd see the, the rough shape and position of an object, but not be able to detect any specific features. After it opens a time. If instead of just completely eliminating activity, you change, it's a pattern. So as I described, the early activity patterns appear to resemble forward motion through space, if instead of having activity patterns that resemble forward motion to space, you make it resemble random activity, sort of, sort of random motion motion. So there's patterns, but it's not consistent pattern as if the animal was moving forward through space, you we can manipulate the circuit so that it's this random pattern. And then when we look at the animals, neural response properties, when it opens its eyes, what we observed is, the animal doesn't detect the neural, the neural system doesn't detect motion. Well, it has sort of primitive motion detection properties, but it doesn't do a good job of detecting motion. So by altering the pattern of activity in the developing rat, not completely eliminating it, but making it random, instead of this forward, motion flow, and then measuring the response properties of neurons in the visual system after the animal opens its eyes, we've shown that this forward motion through space activity pattern is essential for the ability of the animal to detect Well, motion once it does open his eyes.
Nick Jikomes 47:08
Interesting, so so there's a direct relationship between these patterns of activity. And some some I mean, they truly are pre training the circuits to actually do something.
Michael Crair 47:18
That's exactly why because if the, if you disrupt this early pattern activity and make it random, the circuit doesn't form normally, so that neurons don't respond well to motion stimuli once it does open its eyes and it's able to see, so it really does appear to be essential for the development of the normal circuit for visual motion detection.
Nick Jikomes 47:42
So this does I mean, we mentioned dreams earlier, this does remind me of phenomena in the sleep literature where you record from neurons in a particular part of the brain when a mouse or rat is sleeping, and they resemble the patterns that you see when the mouse was actually going through a maze and figuring something out. So it's, it's as if these simulations are happening to train the circuits even in the absence of the direct sensory input from the external world. Is that how you would begin to think about what's going on in a dream, say something like that?
Michael Crair 48:14
Yeah. I mean, that's exactly right. That's it's a wonderful analogy. So my colleague yell His name is Professor George turquois, has studied these early activity patterns in the developing hippocampus, these sorts of gene like patterns of activity, which we think are important for memory consolidation in the hippocampus. And what he shown is that these pre pattern activity in the developing hippocampus sort of dreamlike activity in the hippocampus, at night is necessary for the consolidation of hippocampal memory this place where the hippocampus is responsible primarily for place placed as this memory of where you are in space. These place cell memories are in part driven by dreamlike activity after the animal is done with a sensory experience. And you know, between one day in the next at night, in these dreamlike states, this pattern activity is necessary for consolidating its memory of location and space, which saw the previous day. So this sort of pattern dreamlike activity is a pretty direct analogy to what we're observing in the developing visual system. It's not responsible for memory. Instead, what we're saying is that this early dreamlike activity is mimicking a stimulus which is essential for the development, the self organization of the circuit, so that when the animal opens its eyes it's able to see, instead of consolidating a memory, it's consolidating a circuit that's responsible for vision when the animal opens a touch.
Nick Jikomes 49:55
So I want to talk about human brain development for a little while. So humans As I mentioned earlier, you know, and I think everyone's aware of this humans basically, as far as I know, we have the longest childhood of any animal. And we're generally the least developed animal and the most helpless at birth compared to most other mammals. And more or less, what this means is we've got this sort of super wide window, where there's a lot of plasticity and a lot of room for learning. And the flip side of that is, maybe there's sort of less baked in to our brain compared to other animals. So how would say a human and a mouse compare in that respect? Is it actually true? And how much how much of the human brain would you say is shaped by experience versus how much is already there pre formed.
Michael Crair 50:43
So your description, I think, is pretty accurate. And the the, I think what happens in the human brain is very similar to what happens in a mouse brain. Except there are obvious difference in the human brain. And the obvious differences, there's been a dramatic elaboration of the cortex. So you know, the frontal lobes, and the Pryda lobes are dramatically enlarged relative to a rodent. And so the difference in gestation, and your brain circuit development and plasticity is related more to the difference in the evolution of the brain than it is differences in the, in the in how the brain develops. What I mean by that is, I think the circuits that are responsible for the sort of in the spirit clickers in the visual cortex, the entry level circuits, and the role of this early spontaneous activity, and then the fact that the development is so plasticity, so robust, early in development, and becomes more fixed, is true in the human as well, except for human is so much more brain that's more highly evolved sort of been added on relative to the mouse. And those circuits tend to be much more plastic, and have longer developmental periods associated with them, and are have show robust plasticity in the adult. So I think there's really strong analogies, very similar, except the, the fundamental difference in the human brain in your own brain is that we have so much more higher order cortex, and that stuff is really plastic, and has very prolonged gestation or prolonged development.
Nick Jikomes 52:22
I also wanted to ask you about the concept of critical periods. So the one that is probably most commonly learned in school is maybe what you hear about in a psychology class when you talk about language development in human children. But there's also critical periods in developing sensory systems in mice and other animals. So can you define what a critical period is? And maybe start by talking about we know, from critical period studies in the visual system of mice?
Michael Crair 52:52
Sure. So I mean, I critical periods are an important concept in understanding brain circuit development. They are sometimes misunderstood, because they're, they're thought of as open and shut, like there's a box, the door that opens, and then a door that closes and I think it's, it's much more soft than that. So there's a roll on and a roll off and the plasticity doesn't completely disappear, it just becomes much, much less. So with that preface with that premise, the concept of a critical period for the visual system in a mouse is very similar to a human and so the example in so many actually use the human as a, as a demonstration the visual system critical period. If in a human, you're born with one eye, which is occluded, it might be because you have a physical damage, say to the eyelid, so the eyelid is droopy or closed, you might have a congenital cataract in that eye. So the vision is blurry, it's opaque, because of the thick of the optical effect of the cataract. If you have that visual, you have trouble seeing through that eye. early in development, it if that trouble physical trouble seem to persist after a few years, you lose the ability to see through that. And this is dramatic and easily demonstrated by you can restore the vision in the eye for instance, you can fix the fix the physical damage to the eyelid, or fix the cataract in and this is actually commonly done now in adults. So adults develop cataracts often they're age related. You can have a cataract, you can drop a cataract as an adult, have it for decades, and then have the surgery to repair the cataracts or the optical properties The eyes are restored to normal and you can immediately see through the eye as if you never had lost vision and an eye. Whereas if you have a cataract as a baby you're born with a cataract. Are you very early after birth, unless you fix the optimal use of the eye, within a year or two, you will lose the ability to see normally through that i No matter whether you have years or decades of normal vision, if you don't fix the AI to normal working order early, you lose the ability to see through that. And so that's a critical period for visual circuit development, which is in this case is the first couple years after birth in a human. So this has had a fundamental effect, for instance, for how we treat vision deficits, AB so if a baby is born with some congenital pattern, or it might be the eyes are deviating so that they're looking in two different places at one time, this is sometimes called strabismus, you want to fix a physical and optical properties of the eye. So it's normal so that the neural spits in the brain get wired with normal visual experience. And if you don't fix it right away, within the first couple years, you lose the ability. See, normally through the deviated or the cataract die, even though optically, you've completely restored it. So that's a fixed critical period for the formation of visual response or visual behavior. And the same thing happens in a mouse, it's got essentially the same critical period. So if you blur the vision to one eye, or you deviate the, then you lead to abnormal vision in the deviated eye, or the blurred eye, and that critical period exists in the first couple months after birth for a mouse, and by the time you reach about two months after birth, and that critical period is gone. And deviating or blurring vision in a mouse eye has much more modest or no effects. So that mouse visual system and the neural circuits for visual response parties in that context is very similar to a human, although the timing is completely different, just because development occurs over a different timescale.
Nick Jikomes 57:03
So once those critical periods are have passed, even though there's not like one day where they sharply turn off, and it's this sort of slow rolling and roll out, is it possible to open up some of those, again, pharmacologically say,
Michael Crair 57:17
yeah, so of course, you know, we are we and others, are working hard now to identify pharmacological or genetic or other interventional techniques to restore critical periods. And there have there's been some significant progress in understanding what this shapes the time course of a critical period, and in producing some restoration of plasticity in the adult, but we haven't cracked the nut yet. So, for instance, it appears to be the case in the visual system that the development of inhibitory circuits circuits that are mediated synapses are mediated by GABA synapses, GABAergic synapses, inhibitory synapses in the developing visual cortex, the development of those circuits is really important for shaping the time course of the critical period. And if you delay or prolong the development of those GABAergic circuits, you can prolong the time course of the critical period. And there's some hope that if you could reintroduce some of those GABAergic synapses of those GABAergic neurons in the adult, you might be able to reintroduce a critical region in the adult. So there are a number of attempts to identify what changes over the course of the development to frame the critical period, and reintroduce the developmental elements in the adult to restore the critical period plasticity. And I mean, I think it's very, very promising research. But it's, it hasn't. We haven't cracked the nut yet. We don't, it's probably multiple factors that change. And we may need to adjust to reintroduce multiple factors in order to fully restore plasticity, critical period plasticity seeing the visual system when we adopt.
Nick Jikomes 59:08
So one of the things I've also wanted to ask you about was the relationship between plasticity and the direction you give it through experience, particularly in the context of something like a neuropsychiatric disorder. So for example, a lot of the guests I've had on the podcast have talked about things like SSRIs. More recently, some of the newer results with psychedelics, where it seems in general, like what's happening here is some of these drugs, some more than others, induce heightened levels of plasticity that persist for some amount of time. But the plasticity itself is not intrinsically a good thing. It needs to be directed in one way or the other. So the simple idea here would be that this is what psychotherapy does, it's providing direction whereas the drugs providing this kind of permissive signal for plastic Do you think that's a fair way to think about how drugs that induce plasticity? Should we should think about them? Or? Or is it possible that just inducing or shutting off plasticity can be intrinsically beneficial?
Michael Crair 1:00:13
I, I think in some circumstances, introducing the plasticity can be intrinsically beneficial. But if you, you know, draw on the example of the self organizing nervous system that we see over the course of development, if, if the self organization is misdirected, so the activity which the plastic the activity that's present when the plasticity is reintroduced, is abnormal, or leads to self organization of perturb circuit, it's not helpful. And so not only, not only should one reintroduce the plasticity, but this but the, you know, the activity that is present the training or the, you know, the, the, you know, the therapy that one might undergo, when you introduce a plasticity has to be appropriate in order to train the circuit to a more functional state. So, I think the analogy or the the approach that you described is, is an accurate one, you have to be careful when you introduce plasticity, because you might end up actually destroying or disrupting the circuit, rather than moving it towards a more functional state. So, let's go back to the analogy that I, I brought up at the very beginning, if you have a foundation, and you have a cognitive circuit, which is built on the foundation, if you introduce plasticity into the foundation, and because maybe there's a there's a piece of the foundation that's broken, if you introduce plasticity in the foundation, and the plasticity is such that it just falls apart and melts away, it doesn't do any good to the cognitive circuits, they'll also know the way and they won't function well as well. They won't function better either. So if you're going to reintroduce plasticity into a circuit, especially in the foundational circuits, you better make sure that the activity that's present helps the circuit organize or reorganize itself, in a way which is functionally productive. And that's the sort of, you know, cognitive therapy, which one might undergo or training, sensory experience training or motor training to help the circuit form in a productive way instead of a destructive way?
Nick Jikomes 1:02:29
Yeah, I think that's I think that's an important point, I liked how you put that that, you know, you could have a drug induced a heightened state of plasticity. And that can be directed towards a positive outcome, but it can also be mis directed if it's not if it's not being handled appropriately.
Michael Crair 1:02:44
Exactly. Yep.
Nick Jikomes 1:02:45
So one of the one of the things that you always hear people say, and you know, the number always shifts a little bit, and I feel like the number over my lifetime has always gone up is that the human brain isn't fully developed until whenever, you know, age 25, or something. And this is typically in the context of, you know, talking about some social issue or policy decision, you know, we should or shouldn't allow people to do things at a certain age, because the brain isn't fully formed until some point. Is that true? Does it make sense to even talk about like, a age and approximate age when brain development is over? Is it ever over?
Michael Crair 1:03:23
Ah, yeah, so yeah, it's a very thought provoking question. So I, you know, I like to think that we never stopped developing, I agree with you that, you know, what we learning in the adult is just an extension of the developmental plasticity. So what what maybe, what maybe another way of saying it is that, if we go back to this critical period, distinction is like the, the, you know, the circuits that form are more are most malleable, malleable in certain epics during development. And actually, those are real epics. So if it's too early, the circuit is not malleable, it's not subject to plasticity at all. And if it's too late, it's much less malleable. And I think the sort of cognitive circuits that you're referring to that develop in the frontal cortex, particularly in the in the 20s, they're most malleable in the 20s. That's when those circuits are forming most robustly and are most plastic, and then the plasticity reduces to a degree in the adult. And so it never completely goes away. And I would say the adult plasticity is like a continuation of a developmental phenomenon. It's just the robust plasticity is the most robust plasticity has already passed it by by the time you get say, into your 30s. So I agree with you. I think it's it's maybe and appropriate to talk about, you know, an end to development because one can view adult learning. And plasticity is just a continuation of a developmental phenomenon. And it's just the latest and most robust plasticity you see in the human brain is four cognitive circuits, which are the things that form in the 20s, which lead to things like, you know, planning and the ability to project yourself into the future and anticipate future events. And those sort of cognitive circuits are most plastic have been formed most robustly in your 20s.
Nick Jikomes 1:05:43
Do you think that, you know, stepping away from some of the hardcore science for a moment? Do you think a lot of people have have commented on what I'm about to describe? But do you think it's likely or plausible that as we've developed new technologies, and as you know, even something like COVID, where you've got children now who are not going to school for the length of time that they were? Or maybe you know, they're taking a year where they're essentially homeschooled, you've got things like, you know, our cell phones, which are so mesmerizing, that we're spending, not, not necessarily less time interacting with humans, but certainly a different we're interacting with humans in a different kind of way. As, as we achieve more and more technological process and technology comes into our lives more, is it plausible that the way we interact with technology is shifting the way that the brain is developing and shifting some of these epics of development, such that, you know, the way that the way that the kids are coming up today, or developing today is literally different than the way it was, say for you. In terms of brain development?
Michael Crair 1:06:50
Yes, I think it is. I think it's likely the case, but I don't think it's shifting the time course, necessarily. But it's, it's shifting the outcome. So you know, the, maybe one way to think about it is the kind of typical, typical experience, the sensory and social experience. Children now participate in is different than what I did as a child, right? We didn't have cell phones, and you know, TVs were something you watched for an hour or two, you didn't work you weren't, you didn't have the ability to watch a screen for this period of time that kids do now. And you, you know, you didn't interact with a computer screen, like you do kids do now. So I don't think those differences between what I experienced and what kids do now changed the developmental epics. But what they will do, what they could plausibly do is change the outcome of the development, the time course, what happens over the course of development. So, you know, the circuits that form, the social interaction circuits that form in particular, are probably different now than they were before, you know, screens because the kind of social interactions are different. But I don't think that's necessarily good or bad. It's just different. I don't think one can say whether it's your one could, one could argue that the circuit is adapting to the experience, the animal, the human receives. So it's adapting in a, in a evolutionarily appropriate way, to the experience that a kid now has, it's, but I think it is reasonable to argue that it literally the brains, the typical brain that's forming is different now than the typical brain that formed 50 or 100 years ago, when technology was so very, very different. I just, I wouldn't say it's, it's worse. And I wouldn't say it's better either. It's part of our adaptability as a species that reflects the adaptability of the species. And, and let me just make, you know, go out on the limb a little bit, one could argue that social networks have also changed, you know, as our century or as our experience changes, as our interactions change. And our interactions, for instance, are over the course of computers or the web, or social media, then it likely changes the kinds of social networks that form which are after all, networks of networks of neurons, right? Our brains are networks of neurons and social networks are networks have brain so they're networks of networks of neurons. So the social networks probably have been affected by the changes in the way we interact with our environment in ways which are very hard to anticipate, or even describe. Well,
Nick Jikomes 1:09:47
interesting. Yeah, no, I agree that you can't, you can't automatically say that this type of change in the development of brains given these new social and sensory inputs that we have today is Good or bad? One thing that I think you could say, though, is because the rate of change driven by technology increases over time, the delta between generations in terms of their brain structure is getting bigger. Like if you went back 1000 years or a million years to our ancestors, right? The social and sensory environment that you had compared to your grandparents was more or less identical. Whereas today, it's more or less guaranteed to be very, very different.
Michael Crair 1:10:30
Absolutely true. Absolutely true. So that, you know, the differences in experience, and even differences in nutrition are much more significant now, or the last over the last 50 or 100 years than they were, say 1000 versus 1100 years ago. And so, you know, one can anticipate, yeah, you're right. And so there may be even bigger changes in our brain circuits that form as a result of those large changes in both the experience that people are exposed to over the course of their life, and also, differences in our environment, like nutrition and know the other things like daylight versus nighttime, we're not exposed to circadian rhythms as uniformly now, as we were 100 years ago, when or 200 years ago, years ago, when there was less artificial light. So the lot of things that have changed dramatically. It's hard, but it? Yeah, and it's a great point. Hard to study.
Nick Jikomes 1:11:41
So in general, as a neuroscientist who's been studying brain development for so long, how is your understanding of brain development influenced your general perspective on how you manage your own brain and mental health as you age?
Michael Crair 1:11:54
Ah. So yeah, actually, interestingly enough, I think that the what the biggest impact on brain and mental health, the way that you can most positively impact your brain and mental health is through relatively straightforward means of having great nutrition, exercise, and sleep. And, you know, those are very, very fundamental. Your behaviors, which anybody, hopefully anybody can improve, to, to sustain their mental state, their healthy brain, state, exercise, nutrition, having good nutrition, and good sleep. And, you know, I, I guess, my work over the course of the last few decades, has maybe it's it hasn't fundamentally changed that point. I think what, what my work, and my colleagues, many colleagues work has done as perhaps shown, what the effects of, you know, exercise and good nutrition, and you know, and sleep have on the brain. But the fact that exercise good nutrition and sleep are fundamentally the the ways the best ways to preserve your brain function is it's simple. But it was true and understood 50 years ago, as much as it is today. I mean, the the secondary effect, I would say is that things like continuing to be involved, you know, intellectually involved. So a variety of reading and you know, doing puzzles for fun and interacting socially with people, that sort of constant or ongoing stimulus is essential for preservation of healthy brain function. But I actually think that those are secondary effects to the sleeping and exercise and nutrition that I described earlier. But it's clearly the case that, you know, healthy brain function also depends upon healthy brain activity. And that is, the sort of plasticity or assessments of plasticity sustaining good healthy circuits is important dependent upon activating continuing to activate those circuits in a healthy way.
Nick Jikomes 1:14:58
Yeah, it's amazing how No matter how much we learn how much you read or how much advice you ask, it really just does boil down to the most basic aspects of life, good food, good sleep and good interactions.
Michael Crair 1:15:13
Yeah, that's right. And, and, you know, again, I think that probably goes, there's a strong evolutionary reason for that. So, but, you know, beyond those basic fundamentals, exercise, and sleep and food, you can do a lot to sustain a healthy brain. But don't ignore those fundamentals.
Nick Jikomes 1:15:39
Well, Michael, thank you for your time. This has been fascinating. Are there any final thoughts about brain development, or neuroscience generally, that you want to leave people with?
Michael Crair 1:15:50
Yeah, I mean, I think this is a an amazing time. To be a scientist, it's in particular amazing time to be a brain scientist and neuroscientists, the tools and techniques that we have to dig in and look at what brain activity and manipulate to understand brain activity and brain development are amazing. And they're expanding all the time. And our knowledge of how the brain works. And how the brain develops, is expanding dramatically over time. I think there's lots to look forward to, both in our understanding of the fundamental mechanisms of brain development, and the prospects for doing things like restorative regeneration of neural circuits or, or behavior. If we can understand the basic mechanisms of brain function, and brain plasticity in development. It's a really exciting time to be a scientist. And in particular, it's a really exciting time to study how the brain how the brain works, how the mind works.
Nick Jikomes 1:16:47
Dr. Michael Kreher Thank you
Comments